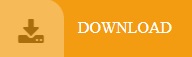

Although some theories posit that attention is mediated by selection very early in the visual pathways, attentional effects have been notoriously difficult to measure using single-unit electrophysiology in area V1 of the monkey brain 7, 8. Shifts in attention are correlated with systematic changes in activity in a number of brain areas. Our ability to perform a visual discrimination task improves when we are cued to attend, without moving our eyes, to the spatial location of the relevant stimulus. One example concerns the role of primary visual cortex (V1) in spatial attention 6. Understanding the relationship between fMRI and neuronal responses is particularly important because some fMRI and neurophysiology experiments have yielded apparently conflicting results. Consequently, this issue has emerged as one of the most important areas in neuroscience.

Although we know that the fMRI signal is triggered by the metabolic demands of increased neuronal activity, the details of this process are only partially understood ( Box 2). The vascular source of the fMRI signal places important limits on the usefulness of the technique. However, the ultimate success of this research depends on the relationship between the fMRI signal and the underlying neuronal activity. This technique is allowing a new era of research, complementary to more invasive techniques for measuring neuronal activity in animal models, to explore the function and dysfunction of the human brain.

fMRI takes advantage of the coupling between neuronal activity and haemodynamics (the local control of blood flow and oxygenation) in the brain to allow the non-invasive localization and measurement of brain activity ( Box 1). It will also be necessary to optimize protocols and analysis techniques if this powerful tool is to live up to its potential.įunctional magnetic resonance imaging (fMRI) 1, 2, 3, 4, 5 has revolutionized cognitive neuroscience over the past decade. It will be important to improve our understanding of the relationships between neural activity, blood flow and metabolism, and fMRI signals, if we are correctly to interpret fMRI studies. There is increasing evidence that this is the case, although the analysis methods used influence the localization of the fMRI signal. The linear transform model also predicts that fMRI responses and neural activity should be colocalized. A direct comparison of simultaneously recorded fMRI responses and neuronal signals in monkey visual cortex found that local field potentials predicted fMRI responses better than multi-unit activity, but that both were variable in accuracy. Studies that have compared neural activity and fMRI responses indirectly have supported this relationship. It should be possible to predict the fMRI response from the underlying neural activity. The frequently observed failure of this prediction could be due to a ceiling effect, or to the disproportionately large responses to very short stimuli that are seen in primary sensory cortex. For example, the fMRI response to a 12-s stimulus should be the same as that to two 6-s stimuli. The linear transform model predicts that the fMRI response should sum over time. The relationship between the signal and the underlying neural activity depends on the fMRI acquisition technique, the behavioural and stimulation protocol, and how neural activity is measured and quantified (for example, by firing rate, local field potential or synchronous firing). It is generally assumed that the fMRI signal is roughly proportional to a measure of local neural activity, averaged over several millimetres and several seconds - the linear transform model of the fMRI signal.
#Hwat does how to#
But what does fMRI tell us? Until we know exactly what the fMRI signal represents, we will not know how to interpret the results of these studies.
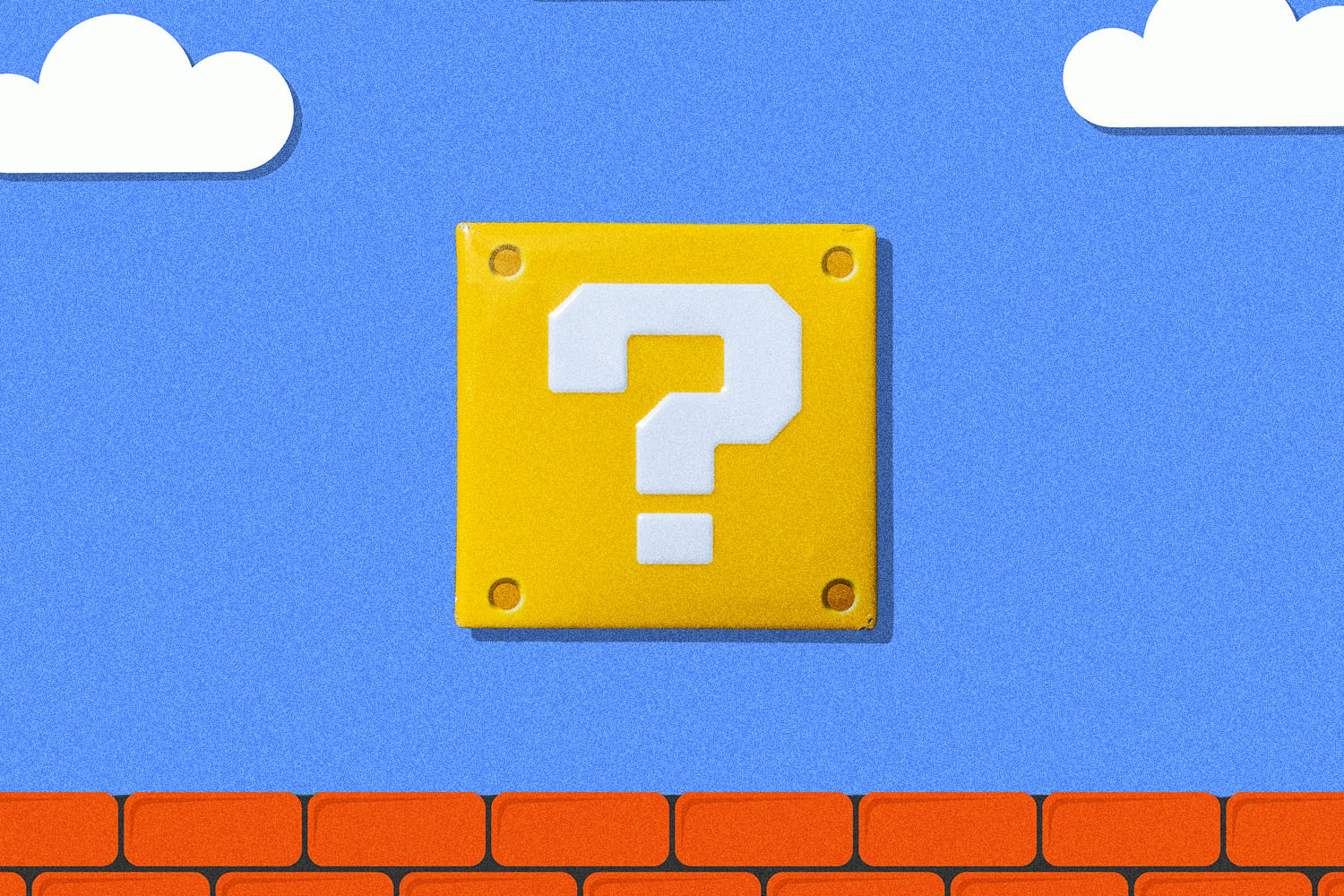
Functional magnetic resonance imaging (fMRI) is now widely used in cognitive neuroscience to look for changes in neural activity that correlate with particular cognitive processes.
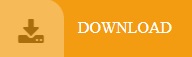